Saving our Skins: Chapter 13. Dark Clouds & Silver Linings
Atmospheric Reflections from a Lauder Star-gazer
The continuing story of “Saving our Skins”. Serendipity in disaster.
Updated September 5, 2021.
In June 1991 an ominous contortion of volcanic ash clouds spewed high into the air above Mount Pinatubo in the Philippines. The volcano finally erupted cataclysmically on June 15 killing more than 700 people and leaving 200,000 homeless. The human cost could have been much worse. If public warnings hadn’t been heeded, the death toll would have been in the thousands. Property damage was in the hundreds of million dollars.
The eruption injected 20 million tons of material directly into the stratosphere. Some went as high as 35 km. Few volcanic eruptions have enough power to penetrate that high, and once in the stratosphere the debris can last a long time. In the lower atmosphere it would be rained out in a few weeks, but there’s no rain in the stratosphere, and no turbulent mixing. The debris is only gradually diluted through gravitational settling and, as Ben Liley later calculated, the smallest particles can remain aloft in the stratosphere for years.
Over the next few weeks, the cloud dispersed to higher latitudes in both hemispheres. We knew from the smaller 1981 eruption of El Chichón in Mexico that our NO2 measurements at Lauder would be affected. But that was before our UV measurements began. We were now also concerned about possible effects on ozone and UV radiation. And this eruption was much bigger. It injected more material into the stratosphere than any eruption since Krakatoa over a hundred years earlier. It would provide a once-in-a-lifetime research opportunity for atmospheric scientists. For us the volcanic cloud would most definitely have a silver lining.
Shortly before the Pinatubo eruption, I’d read of a new balloon-borne instrument designed to measure the optical properties of aerosols from the surface to 30 km. The instruments were called backscatter-sondes.
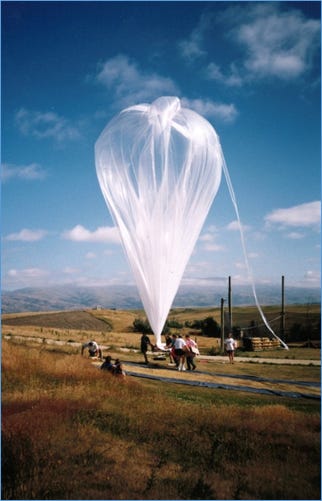
They’re called that because they measure reflected light emitted from lamps on the balloon-borne instrument and then scattered from air in the immediate vicinity back to on-board detectors. The method works because any aerosols in that air increase the size of the backscattered signal. I thought it would be good to use them at Lauder to track any effects of the volcanic aerosols.
I should clarify here what atmospheric scientists mean by “aerosols”, because the term seems to cause a lot of confusion. Spray-cans used to dispense products as diverse as deodorants, hairsprays, and paints are sometimes called “aerosol cans” or just “aerosols”. They contain a gas under pressure, which is used as the propellant. CFCs were ideal gases, as they had a low boiling point, and were stable so they didn’t react with the product to be dispensed. But because CFCs harm the ozone layer, different propellant gases are used nowadays. When you press the release button on these cans, some of the propellant gas is mixed with the product. This mixture is just one example of an aerosol, which is a more general term to describe a mixture of small particles suspended within a gas. Fog, clouds, smog, or the tiny particles left in the atmosphere after volcanic eruptions are other examples of aerosols. They can also affect ozone.
I contacted the backscatter-sonde’s developer, Jim Rosen, at the University of Laramie, Wyoming, and expressed my interest in using them. He was very positive, and we set up a collaboration to launch them regularly from Lauder. We hurried to take advantage of the unique observation opportunities arising from the eruption. Jim’s colleague, Norm Kjome helped us with the first successful launch from Lauder. That was on February 20, 1992, just 8 months after the eruption, and only 4 months after my first contact with Rosen.
That was the beginning of our research program into aerosols (and clouds). My motivation was to understand their effects on the transmission of UV radiation to the Earth’s surface. We wondered if our unpolluted air might be an important factor.
Jim Rosen’s colleague, and later rival, Dave Hofmann, called backscatter-sondes “a poor man’s lidar”. Dave had a soft spot for New Zealand. His first wife was a Kiwi, and he’d often nostalgically refer to Lauder as his “Laramie of the South”. During one of his frequent visits to New Zealand, I introduced him to my sister, Joanne, and they had a brief romance while they were both between spouses. He later became director of one of NOAA’s atmospheric research labs in Boulder Colorado. He was a pioneer of ozone research and remained a good friend of us at Lauder until his untimely death in 2009. It was he who found the funding for us to expand our UV spectrometer systems to Mauna Loa and Boulder.
But he could have had us both killed. That was one afternoon when I was a passenger in his rental car as he drove back to Alexandra from Lauder. At the exit gate from the lab he turned right, directly into the oncoming traffic lane on a blind corner. He’d forgotten that we drive on the left. It was pure luck that there was no oncoming vehicle to end both of our lives.
Aerosol lidars are much simpler than those measuring ozone, with only one laser wavelength needed. The aerosol profile is deduced from the amplitude of scatter from each altitude – as determined from the time-delay between the outgoing lidar pulse and the scattered return signal – and is compared with the amplitude expected from clean air (i.e., from pure Rayleigh scattering). There aren’t many lidars powerful enough to probe the stratosphere. There are only three in New Zealand, and all are at Lauder.
Although simpler, aerosol lidars are more spectacular than ozone lidars, which use UV light that our eyes can’t see. When an aerosol lidar is operating, a pencil of intensely strong green light extends far into the sky. Actually, what you really see is light that has been scattered by air into the direction of your eye. At higher altitudes, where the pressure is lower, the scattered beam becomes much weaker. If the lidar was operating on the moon where there is no air, the beam would be visible only if it reflected off another object, or if you looked directly into it (not recommended).
The collaboration with Jim Rosen and his Laramie colleagues Norm Kjome and Terry Deshler resulted in several useful publications about the evolution and effects of the Pinatubo aerosol, the differences between results from sites in the northern and southern hemispheres, and inter-comparison with other instruments including lidar at this site.
For example, lidar measurements of aerosol profiles are less direct than backscatter sonde measurements, because absorption and scattering losses in the long light path through the atmosphere must also be considered. Assumptions are needed to account for these losses. Lauder, with its clean air was an ideal site to test the methods, and one of our early papers compared profiles from the Pinatubo aerosols measured with both methods and showed that the assumptions used in the lidar retrieval were valid.
That was just one of many studies that turned disaster to opportunity. Another showed that the volcanic aerosols had a direct effect on chemistry in the ozone layer. Working with Makoto Koike, a visiting scientist from Japan, we demonstrated that in the months following the arrival of the volcanic aerosols at Lauder, heterogeneous reactions on their surfaces converted ozone-destroying nitrogen oxides into nitric acid, which plays no part in ozone depletion. That finding was an important step towards understanding the chemistry of ozone loss in Antarctica, where reactions between elevated chlorine levels and nitrogen oxides can also inhibit ozone depletion.
Even if volcanic aerosol does not reach the stratosphere, it can still be a hazard. There’ve been several examples of complete engine shut down in jet aircraft after they have inadvertently flown through aerosol clouds following volcanic eruptions: some with disastrous consequences, and others with miraculous recoveries. We (Ben Liley to be more precise) have helped airlines concerned about safety issues from volcanic eruptions affecting flight paths in our region by providing information about the altitude and amount of aerosol using our LIDAR measurements at Lauder.
Lidars are now the instrument-of-choice for aerosol profile measurements. But even though they have been largely supplanted by lidars, backscatter-sondes remain useful because they give the full profile including the boundary layer extinctions right down to the Earth’s surface. These extinctions in the lowest part of the atmosphere usually have the largest effects on incoming (or outgoing) radiation. The backscatter-sonde measurement is also more direct and easier to interpret because of its shorter more localised light paths.
Aerosols from the Pinatubo eruption had global effects that were seen for several years. They directly blocked radiation from the Sun, reducing the energy transmitted to the surface, and contributed to a temporary reduction in global temperature of around 0.5°C. That finding was the genesis of the idea that global warming could be averted by injecting aerosols into the stratosphere. But, even if that sort of geo-engineering were feasible, there would be potential dangers and possibly unforeseen consequences. For example, it’s now known that “heterogeneous” chemical reactions like those that occur on ice crystals in Antarctica, can also occur on the surfaces of aerosols leading to rapid ozone depletion. In most parts of the globe – but not at Lauder for reasons still unknown – ozone levels temporarily declined for a year or two after the eruption.
We also began continuous measurements of aerosol effects from the ground. Our radiation measurements were expanded to include state-of-the-art measurements of global irradiance from sunlight, as well as its separate diffuse and direct components. A Sun tracker was needed for the direct-beam component. Bruce Forgan, from the Australian Bureau of Meteorology in Melbourne, had developed a radiometer which could also be mounted on the tracker to measure the aerosol optical depth at several different wavelengths. With his help the aerosol measurement program at Lauder was accepted as part of an international network called the Baseline Surface Radiation Network (BSRN), which provided the highest quality radiation measurements available at just a few sites around the world.
Around the same time, we began archiving cloud information obtained from all-sky cameras. The first of these was developed by a recently deceased colleague, Chuck Long, who worked for NOAA at the time. Later it was upgraded to a commercial instrument based on his design. We still use computer programmes that he developed to analyse the sky images to extract cloud parameters.
Our data archives now include daytime all-sky photos for every minute of each day since the turn of the century - including occasional inadvertent selfies, attacks from the beaks of inquisitive magpies, and unwanted deposits from their other end (all of which are interpreted as clouds by the data-processing algorithm). The data archive now includes beautiful high-resolution colour images. The contrasts in brightness in the images clearly illustrate how irradiance can be increased by reflections from clouds that don’t obscure the sun. Overall enhancements over clear sky values can
exceed 20 percent or more.
The amount of aerosol in the air can be characterised in terms of how much it affects the transmission of light. The optical depth of aerosol measures how much of the light would be intercepted and scattered (or absorbed) by the aerosol. Most aerosols simply scatter radiation into different directions without much absorption. But aerosols containing carbon, like soot, are exceptions. They also absorb strongly, so have a much greater effect on the overall transmission of radiation. Clean air is characterised by optical depths for visible radiation of less than 0.1, meaning that 10 percent of the incoming light coming directly down through the atmosphere would interact with an aerosol particle. In Lauder, we found that the optical depth is less than 0.01, meaning that only 1 percent of the light would be scattered.
The aerosol measurements showed Lauder to be one of the cleanest observation sites in the world. In fact, it’s ten times cleaner than what is normally considered clean air in the northern hemisphere, with an air clarity comparable with that at the remote high-altitude Mauna Loa Observatory (altitude 3.4 km) in Hawaii. These low aerosol optical depths are the most important contributor to New Zealand’s peak sun-burning UV being 40 percent more than at comparable northern latitudes. Every silver lining has a cloud!
Another tragedy, this time man-made, affected atmospheric aerosols in a totally unexpected way. Following the 9/11 attacks in USA in 2001, airline fleets were grounded for several days. In the northern hemisphere, a lot was learnt from that opportunistic event. The exhausts from jets provide condensation nuclei for cloud formation. The cessation of flights temporarily reduced cloud cover in the region of major air routes. In turn, the reduction in clouds caused corresponding increases in temperature at the surface below. But, because of our relative isolation and the short atmospheric lifetime of aerosols in the lower atmosphere, New Zealand was not affected.
Apart from the inhabitants of Hiroshima and Nagasaki, the world has so far been spared the horrors of nuclear conflict. Others have speculated on the possible effects of an all-out nuclear war. That would be the disaster of all disasters. New Zealand, being in the other hemisphere (hopefully), far from other land masses (specially far from USA and Russia), would be one of the safest places to avoid the fall-out. The rich and famous realise this and are buying up New Zealand real estate for their personal bolt holes.
During his early years at Lauder, Gordon Keys was involved in research looking at effects of atmospheric nuclear testing by France in the tropical Pacific. His interest back then was on possible effects high in the atmosphere. The effects were of course much more severe lower down, with the devastation of Moruroa Atoll (known then as Mururoa) in French Polynesia. Radioactive fallout from the tests spread far and wide, with disastrous health consequences for some of the personnel involved and international observers, which included New Zealand naval forces. There’s little doubt that there were dire consequences for the inhabitants of other Pacific Islands too. The fall-out often reached dangerous levels in Tahiti over 1000 km from the test site; and effects were even seen 5000 km away in New Zealand. The French were not very popular, especially after their sinking in Auckland of the Greenpeace Rainbow Warrior protest ship in 1985.
But even here there was an unexpected benefit. To assuage their guilt the French Government set up a fund for collaboration between New Zealand and French research. At Lauder we benefitted from that through collaborations with French scientists involved in ozone research, including our long-time colleague Jean-Pierre Pommereau.
In 1993 I was reminded of those French nuclear tests when I met up for the first time with Forrest Mimms III, a private scientist from Texas, at a conference in Kitakyushu Japan. Because of our overlapping interests, we’d corresponded from time to time. He’d invented a simple instrument to measure ozone and aerosol effects on solar radiation. He also used to write a column called the Amateur Scientist for the popular magazine, Scientific American. In one of these he described how to make a simple UV detector using every-day components. An inspirational article for do-it-yourself budding technophiles. But he told me that, sadly, the magazine editors had informed him that they no longer required his services after they found that he didn’t subscribe to Darwin’s Theory of Evolution. I admit that I was surprised at his position on that, given his otherwise impeccable scientific credentials. Perhaps that was part of being brought up in Texas. In any case, I can’t see how that would have a lot to do with his column.
On an outing to nearby Hiroshima just before the meeting, Forrest had used a Geiger counter to measure the radioactivity remaining from the bombing of Hiroshima 50 years earlier. He showed me the data. No measurable effect of the bombing remained. But data from the same instrument showed hugely elevated readings a few days earlier, during his flight from USA to Japan. The readings were a close proxy to altitude. The higher you go, the greater the count rate.
That reminds me of another point of misunderstanding, where some think that the solar ultraviolet radiation that harms our skin is something to do with radioactivity. It’s not. Radioactivity is a property of materials that emit radiation as they decay to other lighter products (e.g., uranium decaying down eventually to lead). During this process they emit particles (e.g., neutrons, protons, alpha and beta particles) in addition to the radiation of photons. That radiation includes shorter wavelengths that are much more harmful than in sunlight arriving at the Earth’s surface, where only the shortest UV-B wavelengths have enough energy to break the bonds holding atoms together in molecules like DNA. In contrast, the shorter wavelength photons (e.g., x-rays) emitted during radioactive decay have much higher energies: enough to completely strip an electron from an atom (i.e., to ionise it) Although still harmful, radiation from the Sun is much more benign because the most damaging shorter wavelengths are absorbed in the atmosphere high above us by ozone and oxygen. The radiation that Forrest detected during his intercontinental jet flight was caused by a combination of gamma rays from the Sun and cosmic rays smashing into the air molecules at those high altitudes.
The atomic bombing of Hiroshima and Nagasaki in 1945 killed around 200,000 of their ordinary citizens. It’ll be cold comfort to their descendants to know that important lessons in atmospheric physics were learnt. As the two mushroom-shaped clouds punched into the stratosphere, they would no doubt have caused big reductions in ozone, but sadly satellite measurements weren’t yet available to record that. On the other hand, the radioactive aerosols from their fallout were perfect tracers to study atmospheric dynamics (winds, etc). A lot would be learnt from the way those aerosol clouds dispersed and settled over the months and years after (there was really no need for Forrest to take his Geiger counter there – the result was already known).
Atmospheric “fallout” from the eruption of Pinatubo half a century later provided another unique research opportunity, an opportunity that was grasped with both hands by the atmospheric research community. The event that was a disaster for some provided a natural laboratory in the atmosphere which scientists used to understand the special chemistry that takes place on surfaces. That knowledge was crucial to understanding the cause of the ozone hole in Antarctica. The surfaces were different - aerosols in one case, ice crystals in the other - but the chemistry was the same. And from the 9/11 disaster we learnt how contrails from jet aircraft increase cirrus cloud, and how that in turn affects climate.
As I revisit this chapter in April 2020, I must sadly report that I haven’t yet thought of any great research benefits accruing from the plague of bushfires over Australia last summer, even though their effects were clearly seen in much of our data from Lauder. Unfortunately, they will only exacerbate global CO2 emissions. It’s been reported that by themselves they released more carbon dioxide than Australia does in a normal year.
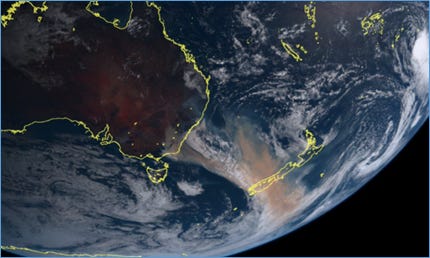
Next week. Tragedy closer to home …