The continuing story of “Saving our Skins”. The beginning of UV research at Lauder …
Updated September 5, 2021.
It was clear that the ozone layer was much more fragile than had been thought before the sudden and unexpected development of the Antarctic ozone hole. Our main concern about ozone is that it absorbs ultraviolet radiation, so protecting us from its harmful effects. Could the ozone losses over Antarctica affect UV in places where people live?
Rather surprisingly, despite all the prior warnings about ozone and its effects, there were few tools available to measure UV radiation. There were only a few simple broad-band meters designed to monitor changes in it, but no concerted efforts to systematically measure the spectrum of UV that reaches the Earth’s surface.
There was huge public concern in New Zealand because of our proximity to the ozone hole. My neighbours and friends - ordinary people, not just scientists - were suddenly interested in my work. It all seemed a bit more relevant than pontificating on arcane atmospheric chemistry. True, a lot of the concern was due to media beat-up. To some extent that was fanned by our own concerns. Only later did it transpire that the concern was misplaced because the Antarctic ozone hole would never spread as far north as New Zealand. Its northern extent is more than a thousand kilometres to the south. The ozone hole typically wanders off the south pole towards South America in late spring, and the only populated regions affected are the southern tips of Chile and Argentina, which are much closer to the pole than any part of New Zealand. In fact, during the time of maximum ozone depletion in the Antarctic spring, there is nowhere on the planet that has more ozone overhead than New Zealand. But at that time, it was not known how far the ozone hole would expand.
When the ozone hole was discovered, only one instrument to measure UV-B was operating in New Zealand. It was a Robertson-Berger radiometer, designed to measure sun-burning UV, that sat alongside the Dobson Spectrophotometer at Invercargill airport. The principal investigator was Reid Basher from the New Zealand Meteorological Service, who was leading a charge to provide UV information to the public. In 1987 he, with colleague Sylvia Nichol, had compared UV levels in Invercargill (46.4°S) with those at the same latitude in the northern hemisphere (Bismarck, ND USA at 46.8°N) and concluded that UV levels were not particularly high in New Zealand. Many years later we were to prove that wrong and showed that peak values were much higher in New Zealand than at corresponding latitudes in the northern hemisphere. It’s just that they weren’t particularly high in Invercargill, which is one of the cloudiest locations in New Zealand. Its low altitude (near sea-level), and high latitude (the southernmost point in the country) mitigate against having high UV.
Paul Johnston and I set about trying to remedy the dearth of good instruments to measure UV radiation by developing a purpose-built spectrometer to do the job. Tony Bittar from the Wellington office of DSIR was also involved. We had made a few measurements of the spectrum of UV radiation at Lauder way back in 1980. He returned with the same instrument in 1988 and we showed that UV levels had not changed appreciably over the intervening period. Although his interest was mainly in solar energy rather than UV radiation, we still learnt a lot from that experience. Our experience developing DOAS instruments to measure nitrogen dioxide was also helpful.
The two main difficulties with measuring UV irradiance are: (1) rejection of light from longer wavelengths in the UV-A and visible regions, which would otherwise swamp the much smaller signals from the UV-B region of interest, and (2) the need for very accurate wavelength registration in the UV-B region where the spectral irradiance plummets by several orders of magnitude over the small UV-B wavelength range from 315 nm down to 280 nm.
To solve the first difficulty, we adapted off-the-shelf double monochromators, rather than the single dispersion monochromators we’d been using for our DOAS studies. In those single monochromators, white light coming in through the entrance slit is dispersed by a diffraction grating into the spectrum that’s focused onto a plane containing the exit slit. At any time, just one colour (or wavelength of light) can get through the exit slit to the detector. That’s why it’s called a monochromator. But by rotating the grating, other colours can be transmitted to the detector, allowing the spectral range of interest to be logged. In double monochromators, the exit slit of the first monochromator also acts as the entrance slit of a second identical monochromator, and the light passed through both is much less contaminated by light from other wavelengths. The stray light from other wavelengths is greatly reduced.
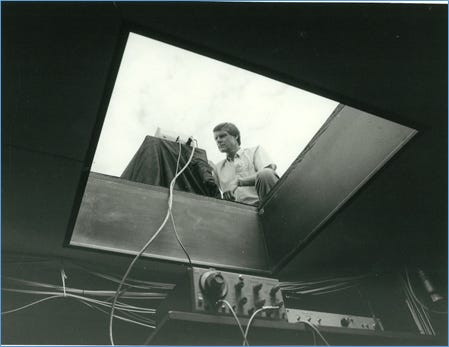
Our earlier experience improving wavelength accuracy needed in DOAS systems to measure trace gases helped us with the second difficulty. In those instruments the signals from NO2 of interest are absorptions of less than 1 percent, which emerge when ratios are formed between solar spectra measured at twilight and spectra measured at solar noon. The Fraunhofer absorption features, from gases in the Sun’s atmosphere, are ten times stronger. We had developed statistical methods to ensure wavelength alignment was good enough to extract the smaller absorptions from the gases of interest in the Earth’s atmosphere from these ratio spectra. There were a couple of iterations, but the techniques we developed in the late 1980s still form the backbone of NIWA’s UV measurements programme today. Long-time UV technician Mike Kotkamp has been responsible for the measurements since the early 1990s. There was a lot of international interest in our work because New Zealand wasn’t the only place lacking decent quality spectral UV data. It was a global issue. I was invited to give talks around the world about our systems, sometimes funded by a group called AFEAS, an acronym for Alternative Fluorocarbons Environmental Acceptability Study. That group was in turn funded by the small group of companies responsible for the manufacturers of the culprit CFCs. There were often a couple of representatives in attendance at these meetings. Among these, Mack McFarland from Du Pont Chemicals was particularly impressive. A great scientist, and great for his
employers as well. He kept everybody honest and on topic.
I recall one such trip, to Gainesville Florida. The travel time took the usual 40 hours from Lauder. I caught a taxi to the hotel meeting venue, where I remained ensconced for the entire week before taxiing back to the airport and flying back home. I did venture out once to the hotel garden where they had a few alligators in a pond. By now several groups were vying for their instrument to be anointed as the chosen one. A group in Canada were extending their ozone-measuring instruments to provide a spectral UV measurement capability. These “Brewer” instruments (named, of course, after their inventor) are still widely used today, but the versions from that period suffered from stray light, and the wavelength range was also too limited. Other contenders were spectrometer systems manufactured by Biospherical Instruments in San Diego. These were very similar to our first spectrometer and had been quickly developed and deployed as part of a network funded by the USA’s National Science Foundation to sites in the Antarctic and Arctic regions, where ozone problems were most likely. Our own NIWA UV spectrometer systems were further improved and received widespread acclaim. Shortly afterwards, a group in Germany, led by Gunther Seckmeyer, also started to make systematic measurements of the spectrum of UV radiation. We formed close collaborations with all those groups. So, concerted efforts to routinely measure the spectrum of UV began only in the late 1980s, and we were among the first.
A big part of our early work was setting up new spectrometer systems to investigate geographic and temporal variability in UV radiation. Over the next few years, as money became more freely available, we took part in several cross-calibration campaigns designed to make sure the geographical variations we reported were not simply artefacts of differences in instrumentation or calibration standards. We hosted a couple of these campaigns at Lauder, but we also took our instruments far and wide including Garmisch-Partenkirchen in Germany in 1994, Nea Michionia, near Thessaloniki, Greece in 1997, and Boulder, Colorado in 1998. To better understand how UV conditions in New Zealand related to other locations, we went on to set up long-term spectral measurements at the high altitude in the USA at Mauna Loa Observatory (altitude 3400 m) Hawaii, and Boulder Colorado (altitude 1760 m); and in Australia at Melbourne and Alice Springs. We also undertook shorter measurement campaigns - lasting a few months or years - in Tokyo, Greenland, and Darwin. Such heady times!
Closer to home, we expanded our network of the simpler broad-band meters that measure sun-burning UV radiation. We upgraded the system at Invercargill and installed similar instruments at Lauder, Christchurch, Wellington (Paraparaumu), Auckland (Leigh), as well as two Pacific Island sites in Fiji and Rarotonga. The locations were designed to complement those targeted by our old DSIR colleague Gerald Smith, who maintained a network of more-troublesome UV sensors for health studies at the main population centres. Shortly after the start of our UV measurements I had taken over from Gordon Keys as the section manager at Lauder, and I remained in that position until 1992 when the National Institute of Water & Atmospheric Research (NIWA) was established. With the formation of NIWA, management at Lauder passed to Andrew Matthews. Andrew was an able scientist who had worked in ozone research since the 1970s at the University of Canterbury, where he completed his PhD. But he was also the “ultimate impresario”, as a German colleague once put it. He was a much better manager than me and was instrumental in raising the profile of Lauder to bring us closer to the wider atmospheric research community. He was also responsible for a quantum leap in the quality and range of instrumentation at Lauder. With his connections and entrepreneurship, he put the place “on the map” for the world’s atmospheric science community and guaranteed its long-term funding. It’s he who should be credited with Lauder becoming the best-instrumented atmospheric research site in the southern hemisphere. Around that time, the world’s atmospheric science community realised that the lack of good ground-based data was a serious impediment to progress. The upshot was that NASA scientist Mike Kurylo led the formation of a new network of highest quality instruments devoted to monitoring long term changes associated with ozone depletion at a small number of sites. It was initially called the Network for the Detection of Stratospheric Change (NDSC). There were to be 5 sites: one at the equator, plus a mid- and high- latitude site in each hemisphere. Andrew made sure Lauder was selected as the southern mid latitude charter site. Our colleague Susan Solomon was by then one of the “gurus” of ozone science. She was based at Boulder, working for the US’s National Oceanic and Atmospheric Administration (NOAA), but had a close connection with Lauder and would sometimes drop in for a visit during trips to and from Antarctica. She spent a sabbatical with us over the summer of 1990-1991 when I was still working closely with Paul Johnston on NO2 measurements. Paul and I worked together with Susan and Canadian colleagues Jim Kerr and Tom McElroy who had been developing a method to derive the “vertical profiles” of NO2 (i.e., its distribution with altitude), inferred from the way the amounts changed during the twilight measurement period. With its low tropospheric pollution, the method worked particularly well for Lauder data, so we adapted it to measuring the vertical profile of ozone and looked forward to applying it to our Antarctic measurements during the ozone hole period. We expected to see the region of famously missing ozone between 15-25 km (as shown in Chapter 9). Sadly, all we saw in the retrieval was a normal ozone profile, just like at mid-latitudes. The method, which is called “optimal estimation” is bootstrapped with an initial guess. The first-guess profile is then “perturbed” by varying it to different shapes, then testing to optimise the statistical fit to the way the data changed over the course of the sunrise or sunset periods. But there wasn’t enough sensitivity in the method to make it useful. I lost interest in it. Similar methods are used in many satellite retrievals, and in ground- based measurements of ozone profiles. I wonder just how useful they really are. Susan did some important work with Gordon Keys at that time, gaining a better understanding of the special “heterogeneous” chemistry of ozone depletion in Antarctica. Because of her interest in Antarctica, she also wrote The Coldest March, a book explaining how atrocious weather during Scott’s last expedition to the South Pole led to his death on March 29, 1912. People asked what we did at Lauder. In a nutshell, we were then working “to understand the causes and effects of ozone depletion”. But, summarising the work I’d been doing with Susan Solomon, I used to tell my son Hamish that I was involved with “the application of matrix inversion techniques for measuring vertical profiles of atmospheric constituents using differential optical absorption spectroscopy.” Much more impressive sounding. I heard him repeat that mouthful just for fun a few times. I’m reminded of the common fallacy in statements like “he was so bright; I couldn’t understand a word he said”. In truth if he was really bright, he would have been much better at putting his ideas into understandable terms.
Scientists from around the world were also starting to recognise the importance of our UV measurements. Not only were we travelling a lot, but others were also coming to Lauder to cross-calibrate their instruments against ours. For now, Lauder was at the heart of the unravelling ozone story.
Next week … Serendipitous results from a natural disaster.