Saving our Skins: Chapter 17. The Montreal Protocol
Atmospheric Reflections from a Lauder Star Gazer
The continuing story of “Saving our Skins”. The most successful international environmental agreement ever.
Updated September 5, 2021.
It sounds like the title of a fiction thriller by Robert Ludlum or John Grisham. True, its wording may not have their immediate excitement, but its real-life impact far exceeds that entire genre. The Montreal Protocol on Protection of the Ozone Layer was signed on September 16, 1987 - now celebrated each anniversary as world “Ozone Day” - and has since been ratified by nearly every country in the world. It came into force in 1989 and has been hailed as the most successful international environmental agreement ever. Its rapid adoption was stimulated by the discovery of the Antarctic ozone hole.
Although we at Lauder can’t claim much credit for its final wording, two New Zealand scientists who later worked for NIWA can. They are Gary Betteridge, who was our DSIR line manager at the time, and Tom Clarkson, from the NZ Met Service. Tom was closely involved in the business end of negotiations because most countries had sent policymakers as their representatives, and he was one of the few there with a first-hand appreciation of the chemistry involved. Gary and Tom were both based in Wellington and though they didn’t talk to each other much, they did work closely with us Lauder scientists in their negotiations.
Prior to the invention of CFCs in the 1930s, the concentration of chlorine (Cl) in the atmosphere was very low, around 0.5 parts per billion (ppb). But by the time the Protocol was signed, it was already more than 1.5 ppb. Still a small amount, but 3 times greater than the natural level. The Antarctic ozone hole first developed around 1980 when concentrations passed the 1 ppb threshold.
The aim of the Montreal Protocol was to bring atmospheric chlorine back below that critical threshold. It would achieve that by curbing the release of CFCs and other ozone depleting gases into the atmosphere. That in turn would be achieved through regulating their manufacture to eventually cease all production of CFCs. A multilateral fund was also set up to allow money to flow from countries that had historically caused the problem (most notably USA and EU members) to developing countries (most notably China and India) so they could transition directly to ozone-friendly technologies, bypassing the older technologies like refrigerators that used CFCs. Unfortunately, in its initial form, the measures introduced were insufficient to reverse the effects of rapidly increasing concentrations of atmospheric chlorine. Only the rate of increase would have slowed down.
But a crucial additional strength of the Montreal Protocol was its establishment of three international scientific watchdog panels, loosely known as the “Science Panel”, the “Effects Panel” and the “Alternatives Panel”. They were respectively tasked with reporting back at regular intervals to the parties of the Montreal Protocol about changes in our understanding of:
(1) The atmospheric science of ozone depletion and UV effects,
(2) Environmental impacts of increases in UV radiation, and
(3) Alternative chemicals that could replace the damaging CFCs.
The scope of these panels was later expanded to include effects of possible interactions with climate change. Because of our early uptake of UV studies at the heart of the problem, and the need for wide geo-political coverage in authorship, I was actively involved in several assessments from the first two panels.
Acting on reports from these panels, the Montreal Protocol was subsequently strengthened in several amendments, named after the cities where the negotiations took place. These included the London, Copenhagen and Beijing Amendments, which brought more ozone-depleting substances under its control and increased the rates of their phase-out. These amendments were crucial to the success of the Montreal Protocol.
Without them atmospheric chlorine would have continued to rise. But with the amendments in place the rate of increase in atmospheric chlorine slowed, and its concentration eventually reached its all-time maximum value of about 2 ppb in the stratosphere around the turn of the century. It has been slowly declining ever since. The decline is slow because the chemicals are very stable in the lower atmosphere, which is what makes them so useful in applications such as foam blowing agents, or propellants, or fire extinguishers.
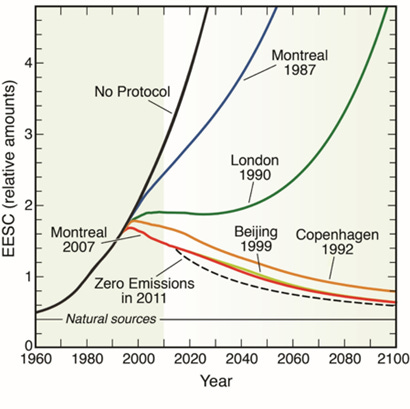
This decline in atmospheric chlorine was achieved initially by replacing the old CFCs with new chemicals (e.g., hydrochlorofluorocarbons, or HCFCs) that had much shorter atmospheric lifetimes, so only a fraction of them survive long enough to be transported up to the stratosphere. As a result, their effect on ozone was only 10 percent of that from the old CFCs. But, with the emergence of higher living standards in India and China, including more widespread use of refrigeration and air conditioning, even these more benign replacement chemicals would have led to further increases in atmospheric chlorine. They were eventually replaced with chemicals called hydrofluorocarbons (HFCs), that contain no chlorine or bromine, and have no effect on ozone. But these replacement chemicals also turned out to have their own problems.
As well as successfully controlling ozone depletion, the Montreal Protocol had also been the world’s most effective tool to date for curbing climate change, because the chemicals that have been phased out are also potent greenhouse gases, with warming potentials thousands of times larger per molecule than CO2. The HCFCs were much better. But not so for the newest HFC replacements. Per molecule, their warming potentials are 50 to 15,000 times more than for carbon dioxide. Interestingly, the most recent amendment (at the time of writing) to the Montreal Protocol, the Kigali Amendment of 2016, had nothing to do with controlling ozone depletion. It targeted the production of HFCs because, although they have no effect on ozone, their warming potential is even larger than the CFCs. New refrigerants are needed.
One of the most remarkable demonstrations of the success of the Montreal Protocol is to consider what would have happened if the agreement had never been signed. This involves modelling studies rather than measurements, which aren’t much use for predicting the future. They can only tell us what’s happened in the past.
These projections of future UV in the world avoided scenario start with atmospheric chemistry models. One such modeller is my colleague, Olaf Morgenstern, a German scientist who moved from Cambridge to Lauder in 2008, but who now works for NIWA in Wellington. I’ve continued to work with him on a few papers since his departure. They use their models to calculate future ozone levels that would have resulted from continued unabated emissions of ozone depleting chemicals, while taking account of all the other chemical and physical changes that occur in the atmosphere. The world-avoided projections can then be compared with projections for the future ozone levels that would occur assuming the gradual reduction in atmospheric chlorine concentrations expected with the continued success of the Montreal Protocol.
The next step is to use another sort of model to calculate the corresponding changes in UV. These are called radiative transfer models (like Sasha Madronich’s TUV model that I mentioned in chapter 15). We can use them to calculate the UVI as a function of sun elevation angle, altitude, and other atmospheric variables. With help from Dan Smale at Lauder,[1] I provided results from this model to calculate the increases in UVI due to the previously calculated decreases in ozone that would have occurred. The results are compelling.
The first of these studies showed the huge increases in UVI that would have occurred throughout the globe. In the second half of the 21st century the UVI at mid latitudes like New Zealand would have been three times larger than it was before ozone depletion began. That would mean that instead of taking 12 minutes for skin damage to occur, the same damage would occur in just 4 minutes. It wouldn’t have been safe to cross the road without sun-protection. While relative increases would have been largest near the Poles, absolute increases would be largest near the equator. By year 2065 peak UVI would have exceeded 45 at the equator, compared with a peak value of about 15 prior to the onset of ozone depletion. Peak UVI values would have exceeded 30 throughout most of the southern hemisphere. While that represents a factor of 3 increase for New Zealand, it’s more than a factor of 10 for Antarctica.
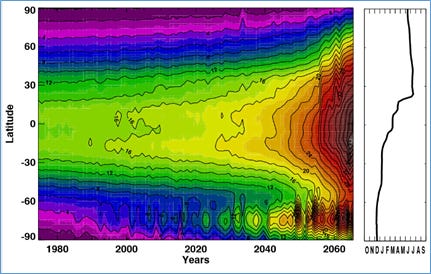
A later study showed that had it not been for the Montreal Protocol, an Arctic “ozone hole” (i.e., a region with ozone amounts less than 220 DU) would already be regularly occurring in the Arctic Spring. This would have been more problematic than in Antarctica because the Arctic is much closer to densely populated areas. They wouldn’t have occurred earlier there in the real world because of the warmer stratospheric temperatures compared with the Antarctic. Rapid ozone depletion in Antarctica occurs on the surfaces of ice crystals that form only below a temperature threshold of about -80°C. Such low temperatures are rare outside of Antarctica. With its geographically symmetric topography, wind-flows during the winter and spring months are dominated by westerlies - without disturbance from underlying mountain ranges. Consequently, there are few diversions of the air to lower latitudes where it can be warmed by sunlight. By contrast, the Arctic’s more complex underlying topography, with its patterns of land-sea stripes, leads to more complex wind patterns. Consequently, the air is regularly transported in and out of lower latitude regions, and so exposed to heating by sunlight.
But if the amount of chlorine in the atmosphere had continued to increase, as it would have without the Montreal Protocol, there would have been rapid ozone depletion in the Arctic as well, especially when effects of climate change are factored in. That’s because, while increasing greenhouse gases lead to increased temperatures at the surface, they also lead to much larger decreased temperatures in the stratosphere. The tropospheric warming occurs because the outgoing energy emitted to space from the Earth is trapped there by greenhouse gases (like CO2, CH4, N2O and others, including CFCs). The warmed atmosphere then re-radiates the excess energy in all directions, rather than just upwards from the surface as would have happened without the greenhouse gases. So, at the same time chlorine was increased, less energy would reach the stratosphere and its temperature would decrease. Those threshold temperatures for rapid ozone depletion, which previously occurred only in Antarctica, could now occur in the Arctic. The Arctic ozone hole that would have by now be forming would be due to the combined effects of both factors.
Even at populated mid-latitude regions, the effects of non-compliance to the Montreal Protocol would by now be serious. The primary motivation for including UV measurements in the NDACC in the early 1990s was to be able to detect long term changes in UV due to long term changes in ozone (depletion or recovery). To achieve that aim, the measurement uncertainty should not exceed plus or minus 5 percent.
For the first few years of UV measurements at Lauder, we did see small increases in summertime UV as the ozone continued to decline before the effects of the Montreal Protocol kicked in. To any physicist, it’s obvious that decreases in ozone will result in increases in UV, all other things being equal. But do all other things really remain equal? For example, changes in ozone could be associated with other changes, such as changes in aerosol or cloud. The sceptics needed to be convinced.
Our results, which were published in 1999, twelve years after the signing of the Montreal Protocol, were the first demonstration that long term reductions in ozone were associated with corresponding increases in UV. Our study looked at peak UVI values, which are the most relevant for skin damage. These peak values don’t occur on cloudless days. Rather, they occur during partly cloudy conditions when the Sun isn’t obscured by clouds, as I explained in Chapter 15.
Singer’s follow-up letter (mentioned earlier), complaining that we had “cherry picked the data”, was typical of the tactics from the Merchants of Doubt. Although there were no repercussions for us, that wasn’t always the case. Their vitriolic and unjustified attacks on American climatologist Mike Mann for his famous (and since verified) “hockey stick” graph of long-term temperature changes in the 2001 IPCC Third Assessment Report on Climate Change is a case in point. The “hockey” Mann referred to is ice hockey, which is a major sport in North America. The descriptor is not so appropriate for New Zealanders more familiar with the field version of the game. They perhaps wouldn’t guess that it depicts the extreme rapidity (in geological terms) of the current increases in temperature compared with those in the past. Much more appropriately, my colleague Ben Liley prefers to describe it as a scythe, reminiscent of that wielded by the Grim Reaper.
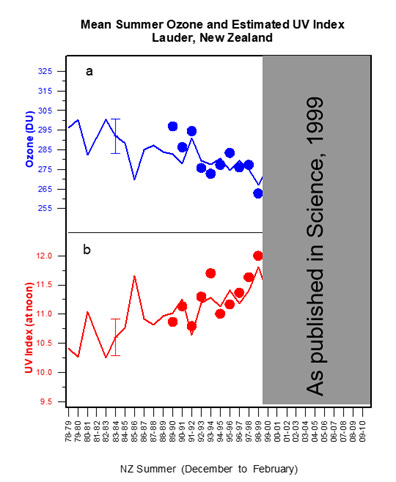
The slanderous comments and personal attacks including trying to have Mann fired from his job made his life a “living hell”, leading to years of litigation and counter-litigation. But the exchange with us showed that even as late as 1999, some were still trying to promote the idea that ozone depletion was not important (reminiscent of the climate-change doubters of today).
But fortunately, the Montreal Protocol was already in place. And working. Atmospheric chlorine reached a peak around the turn of the century and has slowly but surely been decreasing since.
Its success is chronicled in the 4-yearly Scientific Assessments of Ozone Depletion. Each of these tomes contains several hundred pages of densely packed science. But much shorter Executive Summaries are also produced for consumption of non-specialists like politicians. One of the best illustrations I’ve seen summarising the effects of the Montreal Protocol is the four-panel diagram that was produced as part of the Executive Summary of the 2006 Assessment.
There was heated discussion about it. Some thought it was too simple. Scientists are sticklers for detail and accuracy, and some see simplifications like these as “dumbing down” the science. But fortunately the wider perspectives of the panel chairs prevailed. If politicians can understand it, then it should be a doddle to explain it to you enlightened readers. I’ll give it a go.
Each of the four panels in their figure shows changes over the 150-year period from the middle of the 20th century to the end of the 21st century.
The first panel shows the changing annual production of the so-called Ozone Depleting Substances (ODSs). Production rates of the older CFCs (e.g., CFC11 and CFC12) which are most damaging to ozone, increased rapidly until around the turn of the century, apart from a small dip in the 1980s after USA banned their use in aerosol products. At the turn of the century production started to decline rapidly at the same time as the production rate of the more ozone-friendly transitional replacements increased. They were projected to continue their increase until around 2020 when they themselves would be phased out, and replaced by new chemicals such as HFCs which are not harmful to ozone. The name ODS is unfortunate because these gases don’t themselves deplete ozone. They are long-lived chemicals that contain chlorine or bromine that can be released once they get into the stratosphere.
It’s these photochemical by-products, chlorine and bromine that do the damage. The second panel shows their calculated rapid increase until around the turn of the century when the Montreal Protocol began to kick in. Thereafter, their concentrations began to decline, but only slowly because of their stability. Their concentrations are projected to return below the 1980 threshold (when the ozone hole first appeared) in the middle of the 21st century, and finally revert back to natural levels around the end of the century.
The third panel shows the resulting reduction in ozone over the period 1980 to 2000, then a period of relative stability before a projected gradual recovery thereafter as chlorine levels decline. Changes in ozone measured from satellite were also shown to line up with predictions (and have continued to do so). By the end of this century, there is projected to be more ozone than before depletion began, but uncertainties are large.
The final panel shows the calculated changes in UV, which simply mirror the changes in ozone. At the time of that assessment, there was insufficient confidence in UV measurement to include a comparison. The broader spread shown in calculated UV emphasised the increased uncertainty when effects of climate change (e.g., changes in clouds and aerosols) are included. In these global averages, the largest reduction in ozone is only 5 to 10 percent. A beauty of these stylised plots is that the overall message remains unchanged 15 years later, with full recovery expected in the latter part of the century.
At Lauder, after the initial UV increase that we reported for the 1990s, there have been no ongoing decreases in ozone and no increases in UV. In fact, in recent years, the UVI appears to have decreased slightly (as predicted above). In contrast, in the world avoided, UVI levels would already have increased by about 20 percent.
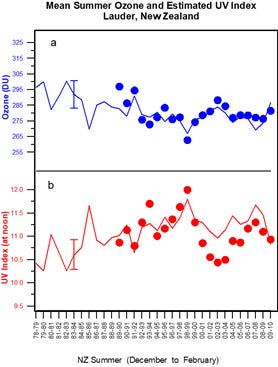
Despite the efforts of Merchants of Doubt like Fred Singer, there was promising progress on solving the ozone problem. In truth though, even before the turn of the century, most of their attention had been diverted to climate change through their efforts to delay action against the fossil fuel industry.
[1] I’m compelled to mention his name explicitly here rather than taking full credit myself because his mum is a friend of ours and a reader of “Saving our Skins” (thereby showing her impeccable taste).
But next week I’ll talk about a few flies in the ointment.