Saving our Skins: Chapter 19. Questions and Answers
Atmospheric Reflections from a Lauder Stargazer
The continuing story of “Saving our Skins”. They started as just a bit of frivolity, but it grew into something far more useful …
Updated September 5, 2021.
I’ve worked closely with Ben Liley in recent years. He occupies the office next door to mine and is now the project leader for NIWA’s radiation studies. I don’t think he’s entirely joking when he summarises our excellent working relationship by saying, “Richard asks the questions and I find the answers”. In one example, I posed the question, “What is the optimum rise in sea level?”, where I facetiously (and perhaps a little defensively) defined the “optimum rise” as that which would render the remaining landmass of New Zealand larger than our “big brother” Australia.
In response, he generated a movie loop, which we now call the Sinking Australia loop – especially when Australians are in the audience. It shows that as sea levels rise, large areas of Australia’s interior are quickly inundated.
For a sea level rise of 300 metres, the continent quickly becomes a disjointed archipelago of islands. With New Zealand’s more mountainous terrain, the overall shape is recognisable for much larger sea level rises. It turns out that the optimum sea rise - by my criterion - is 940 m! Far more than can happen. Luckily too, as it would mean that my home in Central Otago would be nearly 800 m below the surface. Sadly, it seems we will forever be “little brother” to Australia. There’s just one ray of hope. Others have since pointed out that the analysis was simplistic because it ignores the effects of the added weight of water compressing the ground underneath. We know this can be a big effect because the weight of 3 km of ice over Antarctica is sufficient to push the level of ground beneath the ice at the South Pole to below sea level. But I think I’m clutching at straws.
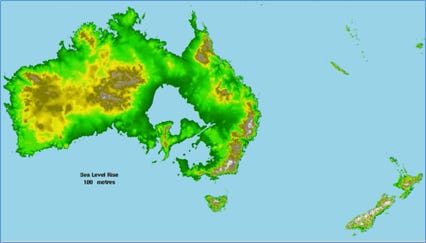
I must make it absolutely clear here that such a dramatic change isn’t going to happen. The maximum sea level rise over the remainder of this century is projected to be less than 1 metre (hopefully much less if we take the right actions). Even if all the world’s ice were to melt, a process that would take thousands of years, the rise in sea level would be less than 100 metres.
In the most optimistic scenarios, sea levels will rise less than 0.3 metres by the end of the century. But to achieve that target, rapid action will be required to curb increases in greenhouse gases. The action that’s needed is currently being forestalled by people like Donald Trump. It’s small comfort to note that their intransigence will contribute to the diminishing value of their family estates as properties less than 1 metre above sea level are inundated.
A less facetious question I posed was “Where on Earth would UV be greatest?”. The question originally arose from an e-mailed enquiry I had received around 2004. I was interested in why anyone would want to know such a thing, so I googled the company, and found that it was a US arms manufacturer. Why would they want to know that, I wondered? If they were concerned about rockets or satellites, then the sky was the limit. Above the Earth’s protective ozone blanket the peak UVI is about 300 - about 20 times more than the maximum at sea level - and damage to exposed skin would occur within 30 seconds (another requirement for astronaut’s space suits).
But I presumed they meant somewhere on the Earth’s surface. I knew the answer would be a high-altitude place near the equator where ozone amounts (outside the Antarctic ozone hole) have a minimum, and where the Sun can be directly overhead to minimise the light-path through the atmosphere. I also guessed somewhere in the southern hemisphere, where overhead Sun can occur at the same time as the closest Earth-Sun separation. Perhaps the tropical Andes? I wasn’t sure exactly where, but I knew they weren’t going to get the information from me for nothing.
I replied that yes, we could find out the exact location, but it would cost them $20,000 (science was well commercialised in New Zealand by then). Strangely (or not?), we never heard back from them. But by then we wanted to know the answer ourselves anyway, so Ben trolled through multiple years of satellite data to find it. In the 2006 NIWA UV Workshop, we presented the result. The highest valid value, was UVI = 25, and it occurred in the grid-cell that contained the city of Cusco, Peru - a most appropriate location, as Cusco was the capital of the old Inca civilisation that worshipped the Sun. On reflection, with NIWA’s charge out rates of around $200 per hour, it probably did cost around $20k for us to do that work. The result was later republished in a book and in a UNEP Assessment, where the world map showing the peak UVI at each grid point was published on the cover of the journal. Unfortunately, in their reformatting to fit the image to the page, the editors truncated the map, so sadly New Zealand did not even appear. Fortunately, it can still be found on our original versions.
The maximum UVI we reported was the average over a 100 x 100 km grid cell that had a mean altitude of 3.4 km. But the highest mountain tops in the region are about 3 km higher, and the clear-sky UVI at these peaks would be about 15 percent higher (giving UVI ~ 29). And when possible enhancements due to reflections from snow and clouds mentioned earlier are factored in, the peak values could conceivably exceed UVI = 35 for brief periods.
We became very popular in South America, as we had put them “on the map” - so to speak. Over the following years, I was invited to give talks in Argentina, Chile and Peru. At one of these, I had the interesting experience of lecturing in English to a Spanish audience via simultaneous translators. Luckily for them, my PowerPoint presentation included diagrams with a few words, but those translators were impressive. I’m not a slow speaker.
Concerning peak UVI, we’ve seen a few published papers that were incorrect, and were an impediment to the advancement of science. We felt a need to correct these wrongs. I can’t think of any better watchdog for scientific integrity than the current peer-review process, where papers that are submitted for publication must first pass scrutiny by peers (normally with at least two reviewers). Any questions raised by the reviewers must, in principle, be addressed to the satisfaction of the editor before publication.
But the process is not perfect and sometimes papers that do not advance the science, or even hinder it, can slip through the safety net. I’ve been motivated to respond to a few of these. Oddly, they often seem to be associated with measurements in South America. But it’s not that the locals are to blame, the authors at fault have been from the USA and Europe.
In one case we were surprised to read a report in 2014 of UVI greater than 43 at a high-altitude site in South America. That was much greater than we thought possible for the conditions. We felt a duty of responsibility to respond to their claim. The paper highlighted both the good and bad side of the peer review process. I had been asked to review an early version of the paper but declined because I worked too closely with one of its authors. It was passed on to others, and following the review comments, the paper was rightly rejected by the journal’s editor. One of those reviewers told me that he later received a request from another journal to review a manuscript with the same title. He again accepted the invitation and found, to his annoyance, that his earlier advice had been ignored. The paper was virtually the same as the earlier version. He repeated his review, and the paper was again rejected by the second journal.
But the problem with the peer review process is that eventually the authors may find a journal with below-par editorial standards, and that’s exactly what happened here. One of the authors of the paper was also an editor of the journal that finally accepted it. To make matters worse, he failed to disclose that he had a financial interest in the instrument that was used. I wonder how many of those instruments were sold because of the ensuing publicity about their “world record” UV.
Our initial rebuttal of their paper showed that the results reported were impossible. Actually, their “record” UVI was the result of an incorrect conversion from a measurement of UV-B that was even more impossible. Their UV-B values were three times higher than plausible. There were many other errors too. Our subsequent analysis of data at Lauder measured with a similar instrument showed that the sensitivity of its UV-B readings to ozone change was far too small, indicating that the instrument wasn’t measuring what they thought. With such a low sensitivity to ozone, we calculated that even if there had been no ozone, the UV-B would be less than the value they’d reported.
In their response, the authors came up with all sorts of wacky ideas to explain their impossible results. One was their assertion that cloud enhancements were greater at UV-B wavelengths than at longer wavelengths. Simple physics tells us that this can’t be true, but theoretical arguments weren’t good enough for them. We used previous research at Lauder by an Austrian post doc Gabi Pfister (who since moved to NCAR) and a Spanish (actually Catalan) student Jordi Badosa, who’s PhD I had co-supervised. Taken together, the studies by Pfister and Badosa clearly showed that cloud enhancements were actually smaller in the UV region than at longer wavelengths. We used those results in a follow-up exposé of their data limitations, and did some further follow-up work to show that even within the UV region, cloud enhancements were larger at longer wavelengths.
Unfortunately, the authors of the original paper still haven’t retracted their result, and if you look up Wikipedia, you may still be directed to their bad data. At the time of writing, the highest values reported from reliable instruments is UVI = 25 (also in the same region). But Ben has assured me that he will one day correct the error in Wikipedia.
Another result that needed a response was a report in 2006 of extreme levels of UV-C radiation measured at 55°S, near the southernmost tip of South America. It was published in the Journal of Geophysical Research, which usually has a high standard of peer review. But not this time. The irradiance they reported in the UV-C (at 250 nm) was larger than the extra-terrestrial value for sunlight, and too large for the observing conditions by more than a factor of 10^15 (i.e., 1,000,000,000,000,000 times too high). In reality none of those wavelengths penetrate the Earth’s atmosphere. Their problem was that the instrument they used was not suitable for the demanding task of measuring very small amounts of UV in the presence of much stronger levels of visible light.
Yet another paper published in an obscure journal in 2018 also claimed to have measured elevated levels of UV-C radiation at Earth’s surface and argued that the 2006 result must indeed have been correct. They had used the same sort of inappropriate instrument as the previous group. We didn’t bother refuting that one, as another critic had already pointed out that their claimed UV-C values were much greater than in unattenuated sunlight before entering the Earth’s atmosphere. To its credit, the journal that published these results lists the reviewers, and makes their reviews available online. I hadn’t heard of any of them, and their reviews clearly demonstrated their incompetence.
I must confess here that I once published an even larger error, though it was perhaps more forgivable. I was reporting our first atmospheric column amounts of nitrogen dioxide in a 1982 paper that was also published in Geophysical Research Letters. The amounts shown in the graph were out by a factor of 6 x 10^23 (i.e., 600,000,000,000,000,000,000,000). In fact, I made the mistake more than once. The same error can be seen in the last figure I showed back in chapter 3 (from a later paper).
I’d mistakenly abbreviated the units of molecules per square centimeter to mol.cm-2, whereas it should have been molec.cm-2. Because mol. is an abbreviation for mole, the difference is Avogadro’s number, the number of particles (6 x 10^23) in one mole (not to be confused with Avocado’s number, but that’s another story).
And here it is, with thanks to Sasha Madronich who passed it on to me. Reproduced without permission:
Q. What is guacamole?
A. No, it’s not what you thought. It’s 6 x 10^23 guacas, otherwise known as Avocado’s number (I don’t often get an audience savvy enough to understand that one, so thank you for listening).
Finally, I want to return to the question of extreme UV at high altitudes. Recall the story in chapter 3 of the epic cross-country journey to the gliding mecca at Omarama. I’d maintained intermittent contact with that community through my father, who spent most of his summers there. But there was a certain historical irony in our group’s re-involvement with them in 2002. The equipment moved there from Lauder twenty years earlier had been associated with measurements of the atmosphere far above our heads, but in the meantime the focus of my UV research had moved right down to the ground. Our new association would be with gliders trying to get back to the upper reaches of the atmosphere (though not as high as the ionosphere).
Our own John Robinson was also a keen glider pilot, and he was approached seeking our assistance by billionaire adventurer Steve Fossett and his protégé Einar Enevoldson. They too were glider pilots, who had assembled a team to attempt the world altitude record for gliders. It was called the “PERLAN” project. Omarama had been chosen as the site for the record attempt because of the favourable winds and mountain configuration that created thermal lift and up draughts – the same motions that led to the formation of lenticular clouds in our valley. Fossett’s team wanted us to provide measurements from our balloon ascents to help them predict the most favourable times for their record attempt. Though a long way from Lauder by road, as the crow flies Omarama is only 50 km to the north on the other side of Mt St Bathans. That meant our data would be highly relevant. In return, they would allow us to mount sensors on the glider to measure how UV radiation changed with altitude. No money changed hands, but John got to help with the deployment as well as developing the detector and electronics.
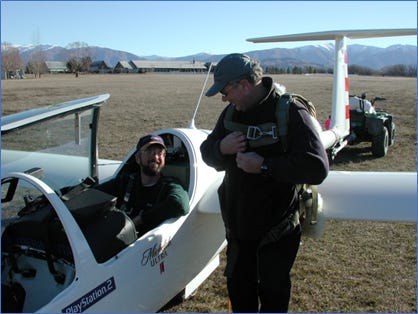
Their target altitude was 90,000 feet (about 27 km). Although Fossett’s lofty goal was ultimately unsuccessful (as was our scientific goal), he did succeed in breaking the speed record in a 500 km triangular flight from Omarama that recorded an average speed of 187 km per hour; and 4 years later his team reached record altitudes near 15.5 km, flying out of a base in Patagonia. Fossett died in a light-plane accident in USA in 2007, but we continued to support Einar Enevoldson in his ongoing record attempts from El Calafate, near 50°S in southern Argentina, where even more favourable gliding conditions for their record attempt were expected. The cockpit windows in the pressurised glider are made of polycarbonate sheet that is susceptible to UV damage, and he was concerned about their breakdown at high altitudes, especially during the period of the Antarctic ozone hole. We helped him answer the question of the UV risk posed under those conditions. At 27 km, the UVI can exceed that anywhere on the Earth’s surface by a factor of three. But their cockpit windows obviously survived any UV damage because in 2016 the group succeeded in breaking their own record. But their maximum altitude was still less than 16 km, well below the peak of the ozone layer, where UVI values were less than half those of their original target altitude of 27 km.
We also got involved in a study with Air New Zealand to measure UV in their aircraft which typically fly at altitudes closer to 10 km. The motivation for that study was to help answer the question of why the risk of death from melanoma was greater for pilots and air crew than for the general public. The risk compared with other groups is measured by what’s called the “odds ratio”. If the risk is the same as for the general public, the odds ratio is 1. Odds ratios greater than 1 imply a higher risk, and odds ratios less than 1 imply a lower risk. Studies had shown that while the all-cause mortality risk among air crews is lower than average, it was much higher than average for melanoma mortality, with an odds ratio of 1.57 for males, meaning their risk of dying from melanoma is 1.57 times greater than for the general public. Most aircraft windows block UV-B, so if UV is to blame it’s probably only the longer wavelength UV-A component. But there are other possible causes too.
The high melanoma mortality rates could also be a consequence of the high levels of radioactivity at flight altitudes (as discussed in chapter 13). Or it could be a behavioural effect. As an aside, that study of odds ratios among different occupation groups showed a couple more interesting results. The highest odds-ratio I’ve ever seen, 33.9, was for death from air accidents (perhaps not surprisingly). More of a surprise was the still extreme odds ratio (14.0 for male cabin crew) for mortality from HIV.
Our study with Air New Zealand is ongoing, and answers to the questions posed will be helped by the development at Lauder of drones and remote-controlled model aircraft for probing the lower reaches of the atmosphere.
A recent study into the susceptibility of microorganisms to UV damage is also relevant here. By virtue of its rather large moat, New Zealand is in the fortunate position of being isolated from the rest of the world, so is not exposed to the full range of plants and animal diseases present elsewhere. For that reason, biosecurity at our borders is taken extremely seriously. But occasionally there are failures, and pathogens arrive, sometimes with disastrous consequences. A recent example was the appearance of a disease called “Myrtle Rust” that first appeared in New Zealand in 2018, with devastating effects on some of our iconic native plants. Our study showed that for many airborne microbes, survival times against damage by UV are extremely short. This means that wind-borne transport of any survivors from other countries (e.g., Australia) to New Zealand is highly unlikely (especially if that transport is at higher altitudes), reinforcing the value of strict border security.
Next week. A bit of light relief …