Saving our Skins: Chapter 8. Zeeman Modulation and More ..
Atmospheric Reflections from a Lauder Stargazer
The continuing story of “Saving our Skins”. Oxford to Pic du Midi Observatory in the Pyrenees and back.
Updated September 5, 2021.
Mike Collins, Lauder’s director in Wellington, had given me a free rein in selecting a research topic at Oxford. His only stipulation was that I should avoid working on the Acid Rain problem. Although that was a hot topic for forest-health in Europe at the time, it wasn’t relevant to New Zealand.
The main focus of the laboratory was measuring atmospheric trace gases relevant to ozone chemistry and climate change. Each of these gases absorbs radiation at its own characteristic wavelengths, so its amount in the atmosphere can be deduced from the unique absorption “fingerprint” that it leaves in the spectrum of sunlight that’s transmitted through the atmosphere. The more of that gas in the light path, the less radiation is transmitted at those fingerprint wavelengths. While a few gases absorb at shorter visible and UV wavelengths, most absorb only at longer wavelengths in the infra-red region.
There were a couple of dozen other post-grad students engaged in DPhil studies. Most of them were working on computer programs that interpreted satellite data to retrieve trace gas concentrations, but I decided against that path. I felt that a hands-on experimental project relevant to ground-based observations would be more appropriate to the limited science budget expected on my return to New Zealand. Because of my earlier interest in the role of nitric oxide (NO) in ozone chemistry, I decided to try to measure that gas from the ground directly, rather than continuing to use nitrogen dioxide (NO2) as a proxy. They had been working already on a method that showed promise.
It’s much harder to measure NO than NO2 because it has no absorption bands in the visible region. The most suitable bands are much further out in the infra-red region, at wavelengths closer to 5 microns. The peak output from the Sun occurs in the visible region, at wavelengths near 0.5 microns (i.e., green light). At longer wavelengths, the energy from sunlight decreases, but the energy emitted by other surfaces at room temperature increases. Their emissions reach a peak at wavelengths near 10 microns. At 5 microns, the energy from sunlight is a tiny fraction of that in the visible region and is comparable with the heat energy emitted from other surfaces at room temperature. You need to isolate the solar signal from that emitted by local surfaces.
I used an esoteric measurement technique called “gas correlation spectroscopy”, where the absorption lines of a gas cell within the instrument are altered so the total absorption through that cell and the same gas in the atmospheric path to the Sun are affected in a way that is proportional to the amount of that gas in the atmosphere. The usual form of gas correlation spectroscopy, which is still used in satellite instruments today, was developed in-house at Oxford by John Houghton and his team. The instrument is called a Pressure Modulation Radiometer (PMR), where absorption lines in the local gas cell are cyclically broadened by a moving piston that changes the pressure.
The new method to measure atmospheric NO would use the splitting of absorption lines by a magnetic field rather than changes in pressure to modulate the signal. This magnetic splitting of absorption lines is called the “Zeeman Effect” named after the Dutch physicist, Pieter Zeeman (1865-1943) who shared the Noble Prize for Physics in 1902 for its discovery. The idea of the new instrument was to periodically vary the strength of the magnetic field inside the gas cell to induce a corresponding periodic change in the absorption by NO. Larger amounts of NO in the atmospheric path would lead to larger modulations in the signal. The instrument would naturally be called a Zeeman Modulation Radiometer (ZMR). Some groundwork had already been done by a previous doctoral student, Hamish Adam, and there was continuing interest in the method from several of the current staff and students.
My task was to further develop the prototype and see if the method worked in practice. My official advisor was Guy Peskett, but I had help from several others, most notably Howard Roscoe, who was a long-time post-doc. Because of absorption by water vapour in the same spectral region, the measurements had to be undertaken from a dry high-altitude site, where those effects would be smaller. We were to take the instrument to l’Observatoire du Pic du Midi, in the French Pyrénées.
After 18 months of development and testing the instrument at Oxford, it was time to put it to the test. With Howard’s guidance I carefully packed the fragile components into a hired van that we would take across the English Channel and drive to the South of France.
With our common rugby heritage, Kiwis are always very welcome in France (unlike their arch-rivals, the English). But this was a particularly interesting time. It was just six weeks after the sinking of Greenpeace’s Rainbow Warrior in Auckland harbour on July 10, 1985. The ship had been actively protesting the continuing nuclear tests in the South Pacific by France, so its government was the prime suspect. Although they wouldn’t admit culpability for the bombing until a month later, most could see the writing on the wall. The people we met were all extremely embarrassed and apologetic wherever we went.
The drive took a couple of days, with the last few kilometres involving a tortuous twisting drive up the mountain. The gear was far too bulky for the téléphérique cable car short cut. The tiny cabins in those days held only 4 passengers. When we finally arrived at the carpark below the observatory, we carefully unpacked the gear, loaded it onto an inclined railway and transported it to one of the observatory domes for reassembly.
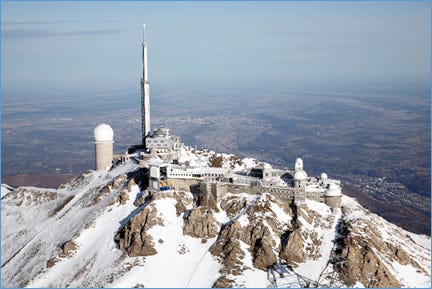
There was plenty of scope for trouble. The gas cell, with its cemented infra-red transmitting windows was fragile and prone to outgassing. Ordinary glass doesn’t transmit far enough beyond the visible into the infra-red, so any windows and lenses were made of magnesium fluoride (MgF2), which does. The gas-filling rig had to be completely free of leaks. Optical alignment was critical. All the electronic components were subject to electrostatic damage, which was much more likely at this high dry site. The state-of-the art infrared detector was made from another exotic material, indium antimonide (InSb), and was not easily replaceable. It was bought from a US military supplier, so may have been developed as a heat seeking sensor to home in on missiles. Because it was a restricted military item, we should strictly have applied for a customs ”Carnet” before transporting it to France and back to the UK. But there was a risk it wouldn’t be allowed, so we didn’t try that.
At the infra-red wavelengths used, all surfaces are constantly emitting heat energy, which would interfere with the signal of interest. Because of this, the detector field-of-view had to be limited to just the centre of the cell window that was fully illuminated by sunlight. The detector and its surrounds also had to be cooled. A vacuum system was needed to maintain the detector cooling for extended periods, and that also had to be leak-free. During testing at Oxford, the detector was cooled with liquid nitrogen, but here only liquid air was available. Liquid air has a slightly higher temperature than liquid nitrogen which has a boiling point of -196°C (77K). And, because of the nitrogen’s lower boiling point, if the container is not vented properly, it can boil off first leaving highly explosive pure oxygen. Also, the detector might have a different response at the higher temperature, and the seals might behave differently. Then there were issues of powering and water-cooling of the magnetic coils. The instrument had to be mounted on an untested Sun-tracker with sufficient pointing accuracy. Finally, all this work was done at high altitude, where brain function is impaired due to lack of oxygen at those low atmospheric pressures. There were boundless opportunities for disaster.
After helping me to set up the equipment, Howard had to depart to nearby Aire sur l’Adour, where the European Space Agency maintained a balloon-launch facility. My measurements had been planned to coincide with a multi-national campaign based there to better understand ozone chemistry. Huge helium-filled balloons would be launched from the site to carry sensitive equipment up through the ozone layer to altitudes near 30 km. On their way up (and down), they would measure vertical profiles of trace gases involved with ozone chemistry to see if their measured concentrations matched predictions. I was left all alone with my ZMR contraption on the mountain observatory.
With great trepidation I fired the equipment up to take my first day of atmospheric measurements at sunrise on the 27th of August 1985. There was no signal on my meters at first, only noise! But that was expected. With the Sun so low on the horizon, no infra-red radiation was getting through. Shortly after 9 am, when the Sun had risen about 5 degrees above the horizon, I was greatly relieved to see the elusive signal start to emerge from the noise. This was the moment that would decide whether my labours over the last two years would bear fruit. Well, not quite the moment. During the day, the instrument would log data to a computer, and only after analysis later in the evening would I know if it had measured anything useful.
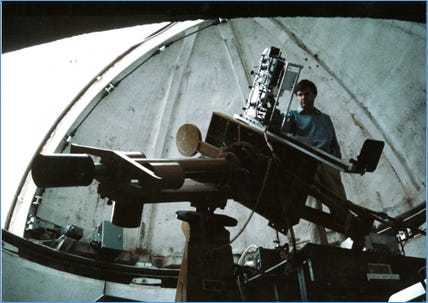
Miraculously, everything worked! A clear signal from the atmospheric nitric oxide we were trying to measure jumped out at me from the data. My DPhil quest was still alive, though a lot of analysis and write-up work was still needed.
Over the next three weeks, the weather was good enough for me to collect 11 days of good data. Each day I was up before sunrise, making measurements from dawn ‘til dusk. Except that we were in France, so each day was punctuated by an extended lunch break, during which quantities of red wine were consumed. The wine was watered down to reduce its cognitive effects, which tend to be more severe at high altitude. For whatever reason, the data quality was decidedly better in the mornings than in the afternoons. Perhaps because of more convective activity, bringing interference from clouds and water vapour, but perhaps not… But aside from that, the measurements were interesting and rewarding. The retrieved amounts of NO were sensible, and the data clearly showed the pattern of diurnal variability predicted by theory.
Abiding memories of the measurements in Pic du Midi included the trip there in a hired van with Howard: with his ever-present guitar and his insistence on keeping a melting pack of over-ripe camembert cheese in the hot glove box of the van. Another highlight was Louise and the kids driving down in our Morris Marina, with 9-year-old Andrew navigating, to make the pilgrimage during the school holidays to nearby Bagnères-de-Bigorre – not far from Lourdes – at the foot of the Pyrénées. We were joined at the camping ground there by Howard, who had come across from Aire sur l’Adour with his friend (later wife, then ex-wife) Anne Ryan, to share my 35th birthday celebration with a specially iced “Joyeux Anniversaire” gâteau. No measurements that day.
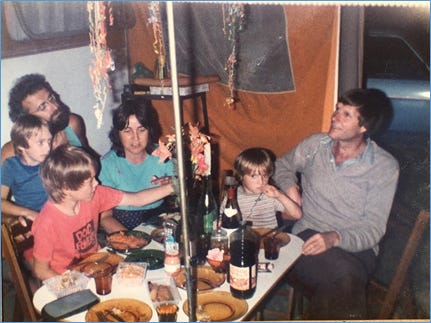
Just two days earlier (September 22, 1985) the French Government had finally admitted planning the bombing of the Rainbow Warrior.
The kids, at least Andrew, also became proficient at walking to the nearest patisserie and asking for “deux baguettes s’il vous plaît”. A bit better than my own French, which was limited to not much more than the phrase “mesure de l’oxyde nitrique”, which you can probably understand without the help of Google Translate.
It had been two years since we first arrived in the UK. I had one year left to complete the analysis and write up the results. I duly submitted my thesis on September 5, 1986. It had the distinction of being the first ever written for the Department using a new typesetting program, Tex – the fore-runner of the much more user-friendly LaTex. David Pairman, another DPhil student from New Zealand, had introduced me to the program but he would submit his own thesis a few weeks later. The professionally bound thesis looked very nice with its maroon cover and gold lettering.
The golden rule for any doctoral thesis is to keep it short so you don’t annoy the examiner by its bulkiness before he or she even opens the tome. By judicious adjustment of line spacing, I contrived to keep mine below 200 pages. But it was heavy-going to read, containing a lot of long words, figures, tables, and equations. They were all beautifully typeset, so for your edification and enjoyment I’ve included a sample.
… and there’s plenty more where that came from. I know any physicists among you will be poring over the equation gleefully, but for most of you I suspect it looks like Greek. That’s because it is. We physicists often use Greek symbols as abbreviations.
The thesis I had produced was - like most academic theses - the antithesis of a “light” read. It was read in detail by myself, my supervisor (I think), Howard Roscoe, and at least one of my external examiners, namely John Pyle, who had been at Oxford, but was by then “down the road” at Cambridge. I’m not so sure about the other examiner, Jim Williams, who was nearing retirement and was more interested in campanology (bell ringing) and ales, and not necessarily in that order by that stage of his lengthy career at Oxford. I must make a pdf copy of it to ensure it’s available for future generations!
Between the time of submitting my thesis and my graduation on October 16, a lectureship position was advertised in the department. The job description seemed tailor-made for me, and Fred Taylor (not tailor) encouraged me to apply. Louise and I thought long and hard about it but decided against putting in an application. By then we had set our hearts on returning to the New Zealand summer. The UK was OK, but it wasn’t home. And there was also the small matter of my NRAC Fellowship. Remaining for a job in the UK would mean repayment of the amount that had been invested in my studies, which by then ran to hundreds of thousands of dollars.
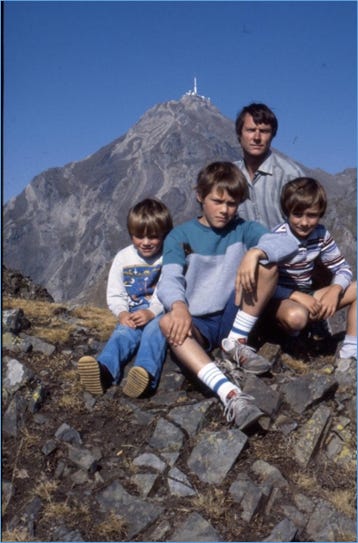
During my time at Oxford, Mike Collins moved up to DSIR Head Office. My new manager, Gary Betteridge, had also encouraged us to move to Wellington when we returned, but we decided against that too. We’d seen enough of city life. But rather than returning to live on-site at Lauder, we’d move back to Alexandra. Much nicer for the family with its schools and other facilities. For the rest of my career, I’d commute the 37 km to Lauder each day in a work van. The commute time was always less than 30 minutes and was never dictated by traffic conditions. Only very occasionally would we be delayed when a mob of sheep was being moved on the road, or by snow or ice on the road during winter.
The ZMR instrument I worked on is now consigned to a footnote in history. I wrote a few papers for scientific journals like Applied Optics about the instrument and measurements from it. My measurements in France did contribute in a small way to validating our understanding of that issue, but my guess is that these are the sum total of all papers ever written on the subject of Zeeman modulation. And the papers about it were probably read by only a few people with nothing better to do with their time.
Interestingly, the international campaign in France was funded by the Chemical Manufacturers Association. That’s the group who make CFCs, the gases that had recently been implicated in possible depletion of ozone. They were actively funding several lines of research into the problem, which was starting to receive a lot more attention. Things had changed dramatically during the planning phase of the campaign.
[1] Ordinary glass doesn’t transmit out as far beyond the visible as 5 microns. So, any windows and lenses were made of Magnesium Fluoride (MgF2). The detector was made of another exotic material, Indium Antimonide (InSb). It was bought from a US military supplier, so may have been used as a heat seeking sensor to home in on missiles. It was a restricted item, so strictly speaking, we should have applied for a customs “Carnet” before transporting it to France and back to the UK. But there was a risk it wouldn’t be allowed, so we didn’t try that.
Next week’s chapter is about the most momentous atmospheric research finding of my time - the discovery of the hole in the ozone layer. The problem with CFCs was no longer just an “academic” issue ….