The continuing story of “Saving our Skins”. This week’s instalment is about the most momentous atmospheric research finding of my time - the discovery of hole in the ozone layer.
Updated September 5, 2021
My time at Oxford University wasn’t just about my thesis work. It’s a hugely exhilarating research environment, with day-to-day interactions with some of the brightest minds in the business (as well as a bit of dead wood). We were at the cutting edge of satellite measurement technology with frequent visitors from NASA and JPL in the USA, and from various research groups in Europe and the UK. Most weeks during term time there would be seminars in the Department, with stimulating speakers from around the world.
The speaker at one of these seminars was a Joe Farman. He worked for the British Antarctic Survey in Cambridge and was a bit of a science boffin, complete with crumpled corduroy jacket. I can’t quite remember if he smoked, but one of those curved Sherlock Holmes pipes would have fitted the image perfectly. And it would have been quite appropriate too, as we were to soon learn.
He’d come across to share some results from ozone measurements that his group had been undertaking in Antarctica. It was most appropriate that he should come to Oxford first with this news. The instrument he was using to measure ozone was called a Dobson spectrophotometer (sometimes shortened to spectrometer) which had been invented in the 1920s by Prof Gordon Dobson who worked at the Clarendon Laboratory at the time and maintained close links with the place until his death in 1975.
His spectrometers were originally intended as a tool to assist weather forecasts. During anticyclonic weather conditions, when the atmospheric pressure is higher than average, there is less ozone overhead. I’ll try to explain why. But first some definitions. The lowermost part of the atmosphere, which extends up to around 10-15 km altitude at mid latitudes, is called the troposphere. There the temperature decreases with altitude, leading to turbulent mixing and convection, causing weather events like rain. You can think of the troposphere as the “weather” sphere. The tropopause is the boundary between the troposphere and the stratosphere above, which contains the ozone layer. Because ozone absorbs solar energy, the temperature in the stratosphere increases with altitude, as it does in any inversion layer. This inhibits vertical motions, so leads to stratification (hence the name), with a local temperature minimum at the tropopause. When tropospheric pressures are high, as during anticyclonic conditions, the tropopause gets distorted and squeezed up to higher altitudes. The ozone-rich air overhead then drains down to places where the tropopause height is lower, such as above cyclonic areas. The amount of ozone overhead therefore gives an independent check on the state of the tropopause and provides clues about future changes in atmospheric pressure and hence weather.
In further recognition of Dobson’s contribution to its study, the amount of ozone in the atmosphere is reported in Dobson Units (DU), where 1 DU is the number of molecules needed to create a pure layer of ozone 0.01 mm thick at standard temperature and pressure (i.e., 1 DU = 2.69 x 10^16 molecules per square centimetre). The global average column amount of ozone is about 300 DU, which corresponds to a layer of pure ozone just 3 mm thick. To turn Churchill’s wartime rhetoric on its head, ”never has a layer so thin done so much for so many” (you may quote me on that).
Dobson’s lab was in the very building where the seminar was to be held. His first wooden instrument, which used photographic plates rather than the electronic photodiode detectors in later versions, still graces a display cabinet in the foyer below. And the office I occupied on its roof was the “New Dobson Hut”. The original “Dobson Hut” was still up there, and I was using it as the base to test my ZMR.
Dobson spectrometers – like our own one at Lauder - remain the gold standard for ozone measurement and are still used to calibrate satellite measurements. There are about 100 of them deployed around the world today. They had been used in New Zealand - intermittently at first - since the 1930s. At that time in the 1980s there was one (instrument #17) at Invercargill, near the southernmost tip of the country, and one of the closest populated areas to Antarctica. That instrument had been in Wellington from 1951 to 1970, prior to its deployment further south, where it was operated by Edith Farkas, of the New Zealand Meteorological Service. But it would soon be moved much further south to Arrival Heights, Antarctica (78°S, 167°E) with a new more automated instrument (#72) being deployed to Lauder. That was the same instrument we talked about in chapter 4.
Joe Farman had come to us to share some puzzling results from his measurements of ozone at the British Antarctic base, Halley Bay (76°S, 25°W), on the opposite side of the continent. The springtime ozone amounts he had seen in recent years had been far too low. He was puzzled because satellite measurements of ozone had been available for several years, having been started in 1978. Yet they had shown no such features. He was a careful back-room scientist, who shunned the public spotlight, and published rarely. He suspected there must have been instrumental problems with his Dobson instrument, but after exhaustive checks, he and his colleagues Brian Gardiner, and John Shanklin, had finally been able to discount that.
Shortly after that seminar, in May 1985 while we were well into planning our campaign in France, they published their landmark paper in the prestigious journal Nature, where they alerted the world to what would become known as the Antarctic “ozone hole”. They even went on to point out that the decreases in ozone were strongly correlated with increases in the concentrations of CFCs, making catalytic cycles involving chlorine rather than nitric oxide the most likely culprit. Even more impressive was their suggestion that the rapid ozone loss may have been something to do with the very cold atmospheric temperatures that are reached only in that part of the world.
Modest depletion of atmospheric ozone from increasing CFCs had been predicted, but the ozone losses they had observed in the atmosphere over Antarctica were far more dramatic than anything that had been foreseen. Later it was found that only there does it get cold enough for ice crystals to form in the stratosphere. A special class of chemical reactions called “heterogeneous” reactions can then occur much more rapidly on the surfaces of these ice crystals than for the usual gas-phase chemistry. But in 1985 this was just a theory, and mechanisms of the chemical processes involved were still unknown.
Farman’s paper had been submitted to Nature in November 1984. At the Quadrennial Ozone Symposium, Halkidiki, Greece a couple of months earlier a Japanese researcher, Shigeru Chubachi, had reported unusual behaviour of springtime ozone at Japan’s Antarctic research station, Syowa Base (69°S, 40°E). He could feel some justification for not getting due credit for discovering the ozone hole. Much later, in 1993, he published a paper tellingly titled “The first detection of Antarctic ozone hole” in which he emphasised the important but unheralded role of his team.
But his earlier paper had focused more on the subsequent rapid increase in ozone in summer rather than on the very low values in spring. He also presented the results as “preliminary”, and without any explanation of possible chemical mechanisms. I think Farman’s paper really did deserve the accolades it received, even though it may not strictly have been the first to note such low ozone values.
Chubachi’s paper may also have gone unnoticed for another reason. English wasn’t as widely spoken in the 1980s, even among scientists in Europe. It would be unusual for atmospheric researchers from far-off Japan to be fluent, so it’s possible that the significance was “lost in the translation”. In those pre-globalisation days, most international travellers kept their translation phrasebooks as near to hand as their stash of local currency (Drachmas for Greece in those pre-EU pre-ATM days).
None of the authors of Farman’s soon-to-be-published paper attended the meeting and they wouldn’t learn of Chubachi’s work until later. Although I was at the meeting in Halkidiki (that was where I finally met John Noxon), my decision to attend was late, and I failed to arrive in time from the UK for Chubachi’s paper – at least that gives me an excuse for not noting its importance at the time. I did however, present a paper about our measurements of NO2 in New Zealand and Antarctica, which turned out to be highly relevant. We had published a closely related paper that year in Geophysical Research Letters, and that was one of just 10 papers cited in Farman’s paper. He cited it to argue that chemistry involving nitrogen oxides was not the cause.
But why weren’t these low ozone values seen in the NASA satellite data? Their newly developed sensor, the Total Ozone Mapping Spectrometer (TOMS for short) was mounted on a polar orbiting satellite from which the downward-viewing instrument could view each point on the Earth below twice a day. With that sort of coverage, how could it miss a feature so obvious that it could be identified from ground-based observations at just a single site?
To measure ozone, the TOMS instrument made use of absorption by ozone in the UV region, as seen in the spectrum of solar radiation scattered from the atmosphere near the Earth’s surface back to the satellite. The more ozone present in the path, the greater the UV absorption. The vertical column of ozone was then derived from knowledge of the angles of the light path from the sun through the atmosphere to the surface, and then back up through the atmosphere again to the satellite sensor. There was plenty of scope for error, so there were checks and balances in the retrieval algorithm to avoid bogus results.
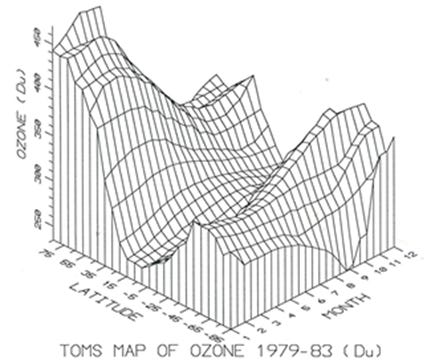
It turned out that the NASA scientists had failed to notice the hole because of a “quality-control” line in their retrieval algorithm that was inserted to ensure that unrealistically low ozone amounts were rejected. The rejection threshold they’d set for “unrealistic” ozone was 180 DU. Any retrieved values less than that limit would be ignored. The sheer enormity of the changes in ozone over Antarctica had caught them out. NASA’s version of the story of course casts them in a better light.
Despite that limitation, an ozone climatology from that time derived from those satellite measurements still showed evidence of anomalously low ozone at high latitudes in October. But the same climatology derived using current retrieval algorithms would show much stronger signatures of springtime ozone depletion in Antarctica, becoming more and more obvious over the following years.
The lesson learnt was salutary in other ways. Although modern satellite data look compelling, often displayed in colourful map projections, the results may not always be accurate. A focus of future research was to be with “validating” – or in some cases “invalidating” - satellite data. Because of Lauder’s pristine location and plethora of instrumentation and expertise in an otherwise data-sparse region of the globe, we would be ideally positioned for this sort of activity. We later uncovered big errors in satellite estimations of nitrogen dioxide concentrations, aerosol optical depth, and surface UV irradiances.
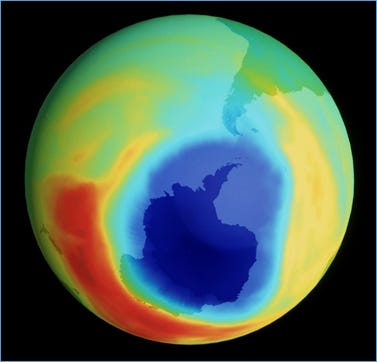
Despite the hiccup at their start, the TOMs satellite instrument and its successors have been hugely beneficial in mapping changes in the size and depth of the springtime ozone hole each year since the early 1980s.
The astounding results from satellite and ground are confirmed by ozone measurements from balloons that ascend to altitudes of 30 km altitude or so. These show that in October, virtually all the ozone between 15 to 20 km over Antarctica can be destroyed, and minimum ozone amounts are sometimes well below 100 Dobson Units, meaning that more than 65 percent of the normal ozone amounts have been destroyed.
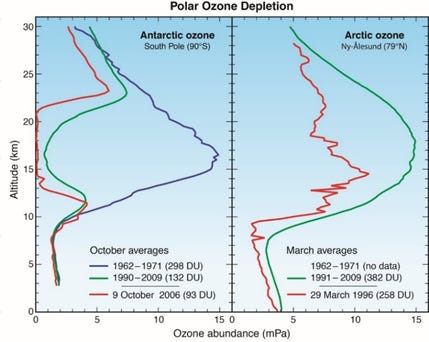
In New Zealand there was just cause for concern. We are one of Antarctica’s nearest neighbours (only the southern tip of South America is closer than New Zealand to Antarctica) and we already had high rates of skin cancer. Could ozone losses in Antarctica extend as far north as New Zealand to increase our UV doses, and therefore our death-rates from skin cancer?
Our friends and family were at the front line.
Next week. Conspiracy theories and the “Merchants of Doubt” …