Saving our Skins: Chapter 2. Sun, Earth and Radio
Atmospheric Reflections from a Lauder Stargazer
Star-gazer work at DSIR Lauder in the early 1980s.
Updated September 3, 2021.
Most people have never seen an aurora, and the likelihood of future generations seeing them is probably diminishing. That’s sad because they’re one of nature’s most spectacular light displays. They dwarf the scale of man-made fireworks. But you can see them only in a few places, and even there only when it’s dark enough for your eyes to become dark-adapted. Then the faint glows can become a majestic display with rapidly moving patterns of light that dance and fill huge quadrants of the night sky.
They occur only over a limited range of high latitudes, mostly in sparsely populated areas. That’s especially true in the southern hemisphere where most auroral activity occurs unseen over the vast but empty Southern Ocean. While tourists can flock to see Aurora Borealis from sites in Scandinavia and Alaska, its southern equivalent, Aurora Australis, is far less accessible. The southern part of New Zealand is one of the few populated areas where you can observe them. They are visible only at night-time, so at high latitudes that means they’re much more likely to be seen on long cold winter nights when most people are snugly tucked up in bed. For townies, even if you’re lucky enough to be outside at the right time and looking in the right direction, you still probably won’t see one. It will be drowned out by stray-light interference from man-made light sources: houses, cars, streetlights, and advertising billboards.
With its clear night skies, and no stray light, Lauder is an ideal location for viewing them. They appear there as faint emissions of mainly red or green light in the southern night sky. Despite our star-gazer efforts to better educate them, some of the locals remained convinced the aurora were somehow caused by reflections of sunlight from Antarctic ice. But, of course, that’s not the case.
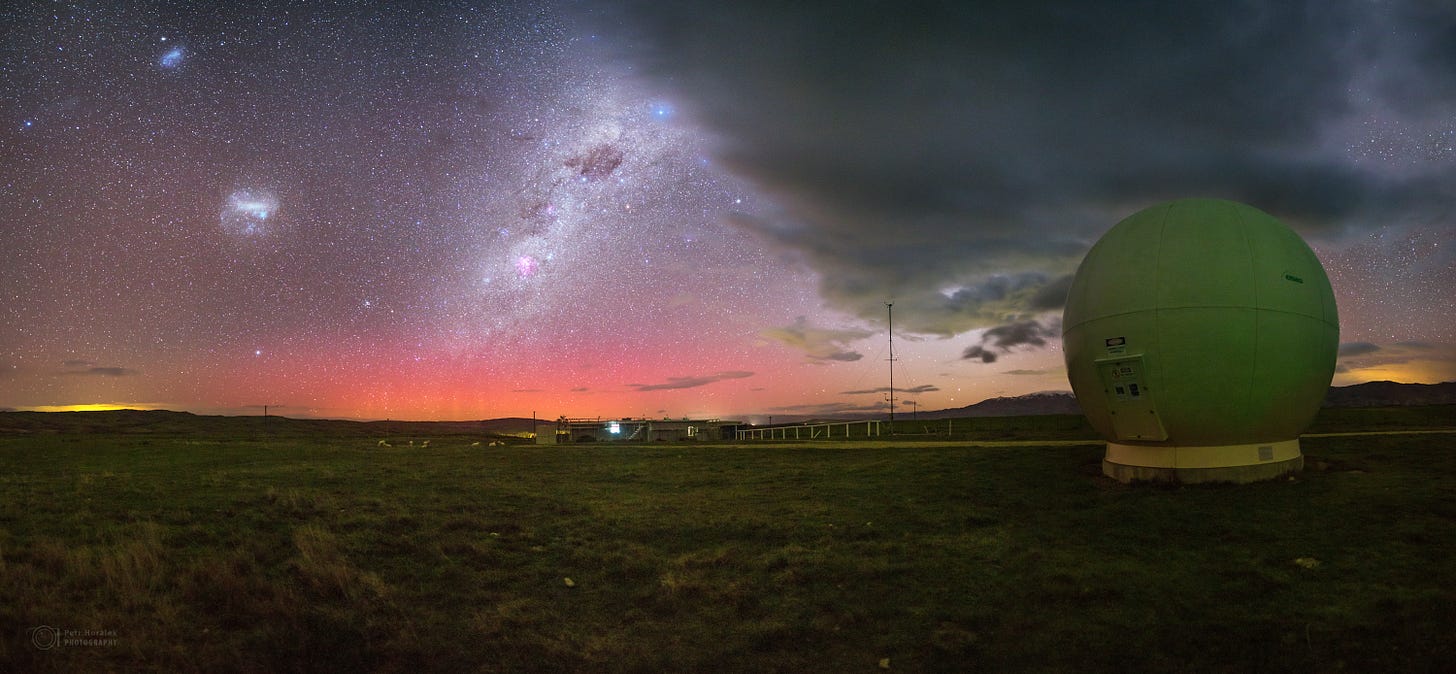
What really causes them, and why do we care? They arise from emissions from the Sun interacting with the Earth’s upper atmosphere. Energy from the Sun arrives mainly in the form of sunlight, which extends beyond the visible to infrared and ultraviolet wavelengths. But this constant stream of radiation isn’t the only thing we get from the Sun. It also emits a highly variable stream of charged particles. These are mainly electrons, and hydrogen ions – which are just hydrogen atoms with their electrons stripped off by the extreme temperatures in the Sun’s atmosphere.
The movement of these charged particles through space from the Sun to the Earth is called the “solar wind”, which travels through space much more slowly than light. Whereas it takes about 8 minutes for light to get here, it typically takes a few days for charged particles in the solar wind. They can arrive at any time of the day or night and can collide with gas molecules high in the Earth’s atmosphere at altitudes from 100 to 400 km. These collisions cause the bursts of light that we call aurora.
But long before they enter Earth’s atmosphere, the charged particles are deflected by its magnetic field and ducted into a small range of latitudes known as the “auroral oval”. These aren’t the usual geographic latitudes defined by the spin axis of the Earth, which is centred on the geographic North and South Pole. Here I mean the latitude with respect to the magnetic poles. These migrate slowly over time and the southern geomagnetic pole is currently thousands of kilometres from the geographic south pole. In fact, it’s not even on the vast Antarctic continent. It lies just off its edge, directly south of Adelaide, Australia (near 64°S, 137°E). So, although Lauder’s geographic latitude is 45°S, it’s about 5° closer to the south geomagnetic pole, with a geomagnetic latitude around 50°S. This brings it close to the edge of the auroral oval, which is centred around 70°S magnetic. So, the southern aspect of Lauder was important.
Before the move to Lauder in 1961 the group had been based at Awarua and Bluff Hill, near Invercargill. But, although further south, those cloudier skies were less conducive to optical studies of the aurorae. When I arrived in 1979, while most of the work was being done at Lauder, the group was still measuring auroral effects on radio waves from a site about 200 km further south at Slope Point, the southernmost tip of the South Island (not quite the southernmost point of New Zealand, as I was emphatically reminded once when showing some visitors around Lauder. They were from Stewart Island, which lies well to the south). The Lauder group was also involved in measurements from New Zealand’s Arrival Heights Observatory near McMurdo Base in Antarctica, another 3,500 km south.
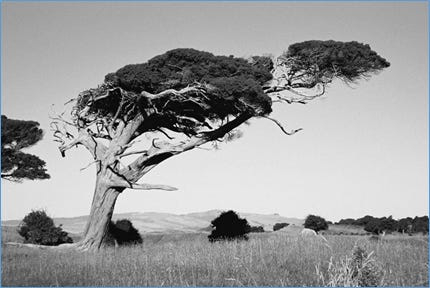
Auroral activity varies on an 11-year cycle in phase with changes in the Sun’s output, as measured by its number of sunspots. In 1979 we were approaching a sunspot maximum. The spectacular aurorae occurring then were part of the motivation for that ill-fated flight over Antarctica. As I write this, chartered flights to Antarctica have resumed, to get a bird’s eye view of the aurora. But that activity may wane over the next few years as we descend from the last solar maximum in 2013 towards a sunspot minimum. As an aside, there’s been speculation that solar activity may be much lower over the next few cycles. If so, this would temporarily inhibit auroral activity, and possibly slow down global warming for a few decades as well. The last two extended periods of low sunspot activity (during the Maunder minimum from 1645 to 1715 and the Dalton Minimum from 1790 to 1830) were associated with lower temperatures, at least in Europe where most of the observations were available.
In the decades before the 1980s, there was intense interest in the aurora because of their effect on both radio communications and navigation. Here’s how that works. At the high altitudes involved, UV radiation from the Sun can break apart the molecules of nitrogen and oxygen in the air, to produce atoms of nitrogen or oxygen. When two of these like atoms collide, they recombine to their original molecular forms, but if unlike atoms combine, they form nitric oxide. But in the rarefied air above 100 km, collisions are less frequent, and the atoms can persist for extended periods. When a charged particle from the Sun collides with one of these atoms in the upper atmosphere, some of the energy can be transferred to “excite” one of its electrons to a higher energy state. The auroral lights are bursts of emitted energy when the electrons in these atoms return to their ground state.
Sometimes, though, the collision has enough energy to completely dislodge an electron from the atom, leaving a positively charged ion and a free electron. This can affect radio reception. Because radio waves travel in straight lines, they must rely on reflections to get global coverage. In those pre-satellite days, the only possibility was to use reflections from the layers of electrons in the upper atmosphere. But that reflection efficiency, and therefore radio reception, is affected by the electron density. Also, as these charged particles continue to move, the electric current so formed affects the Earth’s magnetic field. That in turn, along with the current geographic location of the magnetic pole, affects the pointing direction of compass needles, which at that time were still the prime tool for navigation. GPS navigation systems were yet to be invented.
Back in my undergraduate days at the University of Canterbury in the early 1970s, I had been fascinated by these Earth-Sun interactions. It was probably the only part of the physics course that really appealed to me. I’m reminded of that by my well-thumbed and heavily annotated copy of John Radcliffe’s “Sun, Earth and Radio” that still sits on the bookcase beside my desk.
My honours-year project there, which investigated a late 19th century method of colour photography pioneered in France by Gabriel Lippmann, was also relevant to my new job. The method involved focussing the scene of interest down on to an old-style photographic plate floating on a mercury bath with its photographic emulsion side down. The mercury bath reflects the light back up through the plate, and interference between the incoming beam and the reflected beam causes a layered pattern of light intensities within the emulsion. After the plates are developed, the interference fringes inside the emulsion can reproduce the original image when the plate is re-illuminated with white light. The work required my confinement in a darkroom for prolonged periods, breathing air that was probably contaminated with poisonous gases vaporised from the mercury tray, which was open to the air. (That could explain a lot because Mercury poisoning symptoms include muscle weakness, poor coordination, numbness in the hands and feet, skin rashes, anxiety, memory problems, and trouble speaking, hearing or seeing!). The Kodak-Eastman colour photography method that evolved in the 20th century (since superseded by digital methods) uses a completely different principle, but the skills in optics I learnt from that study were to prove valuable in my new job.
At the time I arrived at Lauder, the era of monitoring the planet using satellite-based instruments was in its infancy. Typically, the satellites are launched into orbit about 1000 kilometres above the Earth’s surface, where there is so little air that frictional losses are low. If they are launched to travel with just the right horizontal speed, they can keep orbiting the Earth indefinitely. The speed required to maintain the orbit at these heights means that the satellites circumnavigate the Earth in a few hours. For full coverage of the globe beneath, a north-south orbit path is needed, but more typically, near-global coverage is achieved by tilting the orbit by a few degrees from the North-South direction. Then, as the Earth spins on its axis below, instruments on board the satellite can build up daily snapshots of atmospheric, ground-surface, or ocean-surface properties.
Some of these instruments measure infrared radiation (heat energy) emitted from the Earth’s land or ocean surfaces. At first, the satellite programmes were mainly run from the USA by NASA and NOAA. Early examples were the LANDSAT and SEASAT programmes, which provided the first global patterns of surface temperatures. I’d been using SEASAT data in my master’s thesis.
Other satellite-borne instruments made use of reflected sunlight to measure the concentrations of atmospheric gases – like ozone - that absorb radiation at some wavelengths. Some detected direct emissions from the atmosphere. Of particular interest to the team at Lauder was the series of International Satellites for Ionospheric Studies (ISIS) maintained by Canada. These measured auroral activity from 100 to 400 km altitude in both hemispheres. At the time these instruments were developed, there was insufficient on-board satellite data storage to allow data accumulation over a full orbit. Ground stations were required around the world to receive the data, write to tape, and send it back to Canada. Lauder had recently been confirmed as one of these sites. A decision that would secure its future for the next few years.
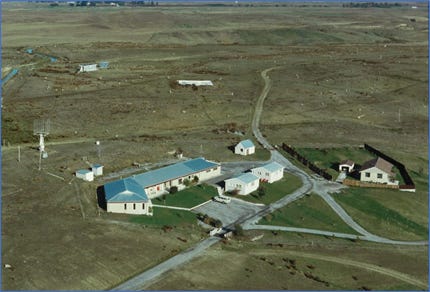
We’d rushed from Suva to Lauder - leaving my students’ final exam scripts to be marked by my colleagues – so I could rub shoulders at Lauder with a visiting luminary (if you’ll excuse the pun) of upper atmosphere research, a Professor Mike Gadsden. He’d worked there in the 1960s but was now on sabbatical leave from Aberdeen University. My rush to work with him was a bit of a waste of time. I was just 29 years old with no special training in the field. He was singularly unimpressed with my ignorance on this new subject area for me that had been dear to his heart for decades. After he left, my next jobs there were to do with measuring faint (sub-visual) emissions of light from altitudes around 400 km.They are called Stable Auroral Red emissions (or SAR arcs). To this day I don’t know why we bothered with them.
The next instalment, “Changing of the Guard”, discusses the seismic changes in work at Lauder, beginning in 1979. (2540 words).